the Creative Commons Attribution 4.0 License.
the Creative Commons Attribution 4.0 License.
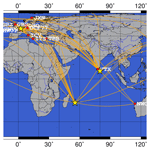
A VLF/LF facility network for preseismic electromagnetic investigations
Patrick H. M. Galopeau
Ashanthi S. Maxworth
Mohammed Y. Boudjada
Hans U. Eichelberger
Mustapha Meftah
Pier F. Biagi
Konrad Schwingenschuh
Earthquakes are one of the most frequently occurring natural disasters. Many indications have been collected on the presence of seismo-ionospheric perturbations preceding such tragic phenomena. Radio techniques are the essential tools leading the detection of seismo-electromagnetic emissions by monitoring at very low-frequency (VLF, 3–30 kHz) and low-frequency (LF, 30–300 kHz) sub-ionospheric paths between transmitters and receivers (Hayakawa, 2015). In this brief communication, we present the implementation of a VLF/LF network to search for earthquake electromagnetic precursors. The proposed system is comprised of a monopole antenna including a preamplifier, a GPS receiver and a recording device. This system will deliver a steady stream of real-time amplitude and phase measurements as well as a daily recording VLF/LF data set. The first implementation of the system was done in Graz, Austria. The second one will be in Guyancourt (France), with a third one in Réunion (France) and a fourth one in Moratuwa (Sri Lanka). In the near future, we are planning to expand our network for enhanced monitoring and increased coverage.
- Article
(2954 KB) - Full-text XML
- BibTeX
- EndNote
Earthquakes (EQs) are one of the most dangerous and unavoidable natural disasters. Large-magnitude EQs can cause casualties and damage property. EQs are ruptures along faults caused by a sudden release of energy after stress accumulations in the Earth's crust, i.e., in the upper part of the lithosphere. The major regions of EQ occurrence are (a) the Atlantic–Indian Ocean ridges, (b) Pacific regions including southeastern and middle Asia, (c) the Middle East, and (d) southern Europe. The seismicity is linked to the tectonic activity of the Earth: the large-scale convection currents in the mantle lead to relative displacements, slow deformations and stresses in the lithosphere, which result in episodes of rupture or subduction that are sources of earthquakes of various magnitudes. A map of the distribution of the seismicity all around the world, for magnitudes M≥4, is given by Keilis-Borok (2002, Fig. 1). Most EQs are considered weak, and hence the life on solid ground does not get affected by these events. Under specific conditions, they can cause tsunamis, which can be disastrous. Therefore, it is of utmost importance to the welfare of society to predict them to save lives and to minimize the damage.
During the past few decades, important progress has been devoted to the quest for earthquake precursors (e.g., Cicerone et al., 2009; De Santis et al., 2019) and more particularly to the study of seismo-electromagnetic precursors. Recent reviews by Parrot (2018) and Hayakawa (2015) emphasize the- space and ground-based observations that cover a large spectral domain, from a few hertz to several hundred kilohertz. Investigations of seismo-electromagnetic emissions allow for the provision of microscopic information on the lithosphere before earthquake occurrences. In addition, such electromagnetic waves can propagate over considerable distances in the lithosphere. These two properties, i.e., precursory occurrence and long-distance propagation, are fundamental for short-term EQ prediction (Hayakawa and Hobara, 2010).
In this work, we consider electromagnetic (EM) precursors observed in the very low-frequency (VLF) and low-frequency (LF) bands by electric field experiments on board satellites or by ground stations. One important feature of the seismic EM precursors is the study of ionospheric disturbances observed above earthquake regions. The basic method consists of analyzing amplitude and phase variations related to the propagation of the transmitter's sub-ionospheric VLF/LF signal. Usually the transmitter signal is mainly reflected by the ionospheric D- and E-layers and detected by ground stations. Hayakawa et al. (1996) proposed the most confident indication of seismic ionospheric disturbances using the so-called terminator time (TT) method. The authors showed in the case of the Kobe EQ on 17 January 1995 a significant shift in time of the diurnal variation (amplitude and/or phase) around local sunrise and sunset. Later on, Yoshida et al. (2008) suggested that the shift in time is due to an occurrence of interference between ground and sky waves. This interpretation of the TT-observed shift leads to evaluation of the ionospheric height. In addition, the TT method has been applied to derive the VLF modal interference distance by Samanes et al. (2015) using a long-term database (2007 to 2011) recorded by the South American VLF Network (SAVNET, Raulin et al., 2009). This distance is estimated from two successive TT minima of VLF transmitter amplitude linked to nighttime wave propagation in the Earth–ionosphere waveguide. This study allowed deriving the undisturbed height of the nighttime waveguide estimated to be of the order of 88 km. Moreover, seismic ionospheric disturbances are found to exhibit particular behaviors when investigating the trend, dispersion and nighttime fluctuation of the NAA transmitter (located in Cutler, Maine, USA) as reported by Hayakawa et al. (2011). The authors found that about 12 d before the 2010 Haiti EQ (magnitude 7.0 and depth 10 km), there was a noteworthy reduction in trend with simultaneous intensifications of dispersion and nighttime fluctuation. Moreover, Earth's tide effect was mentioned about 2 months before the main shock, which can only be considered for a huge EQ.
Additionally, several investigations showed a drop in the amplitude of the transmitter signal several days before earthquake occurrences using different methods in the treatment of the radio signal like the wavelet (e.g., Biagi et al., 2019) and spectral techniques (e.g., Boudjada et al., 2017). Similar studies also show a reduction of the VLF signal phase noise during the preparatory time of EQs (e.g., Nina et al., 2021). In the model by Molchanov et al. (2006) the preseismic ionospheric disturbances are linked to an upward energy flux of atmospheric gravity waves generated by the EQ preparation zone. The relationship between the radius of the earthquake preparation zone ρ and the earthquake magnitude M is given by ρ[km] =100.43 M (Dobrovolsky et al., 1979). Bowman et al. (1998) obtained a similar relation.
In the following, after a presentation of the scientific objectives, we describe the VLF/LF reception system and give an example of observations. Then we discuss the relevance of the sites chosen for the antenna setup.
The main scientific objective is the detection of seismic EM precursors derived from the amplitude and the phase of the VLF/LF transmitter signals. Therefore the seismic preparation zone should be localized between the transmitter and the reception stations within an area given by the Dobrovolsky radius. Solar and geomagnetic activities contribute to the disturbance of the sub-ionospheric VLF/LF wave when it propagates in the waveguide between the Earth's surface (ground or seawater) and the ionospheric D- and E-layers. The propagation conditions, in particular in the D-layer, may be affected by natural ionospheric currents, which has the effect of modifying the amplitude and the polarization of the wave. Resonance phenomena can also appear at particular frequencies (Maxworth et al., 2015). As a consequence, we need a better categorization and characterization of the electromagnetic environment surrounding the VLF/LF reception station.
The VLF/LF system we plan to implement will consist of a monopole antenna equipped with a preamplifier, a GPS receiver and a sound card to digitize the received signal. This device will be identical in all respects to the VLF/LF reception system set up at the Space Research Institute in Graz (Austria) in order to study earthquake precursors as the major scientific objective. Figure 1 displays a schematic of the system.
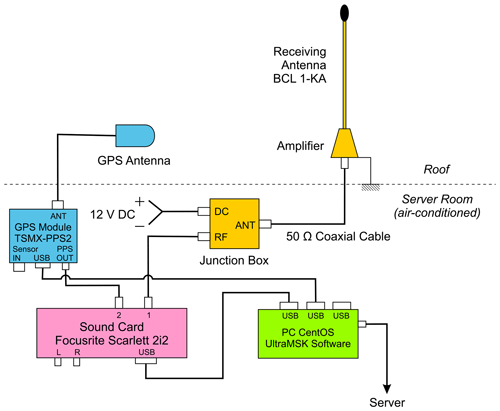
Figure 1Block diagram of the reception system including the monopole antenna, the preamplifier, the GPS receiver and the sound card.
The receiving antenna (Procom BCL 1-KA) will be of monopole type (length 92 cm), omnidirectional, vertically polarized and designed for a frequency band 10 kHz–100 MHz. It is equipped with a preamplifier that must be connected by a 50 Ω coaxial cable to a junction box that separates the DC current from the 12 V power supply and the RF signal. The radio signal will then be sent to a sound card to be digitized. The model used is Focusrite Scarlett 2i2 with a chosen sampling frequency of 192 kHz and a 24-bit digitized output. A GPS receiver module with a PPS output (pulses per second) allows precise synchronization of the radio signal thanks to the minimal jitter. Finally the output of the sound card is connected (by a USB cable) to a PC running under a Linux operating system (CentOS). Specially dedicated software, called UltraMSK, will lead the recording of the detected transmitter signals and deliver a daily data file. Figure 2 displays photos of the new reception system. UltraMSK is a software app (radio receiver) in a particular hardware configuration (Clilverd et al., 2009) capable of measuring the amplitude and phase of VLF/LF radio transmitters as detailed at https://www.ultramsk.com (last access: 15 November 2023).
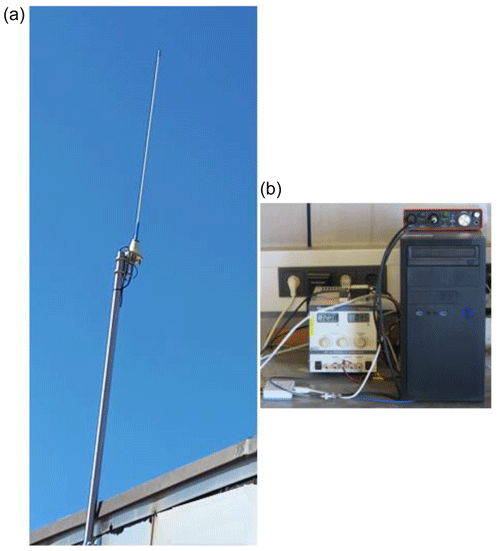
Figure 2Photo of the new reception system in operation since 2019. Panels (a) and (b) display the new antenna (i.e., Procom BCL 1-KA) rigidly fixed on a pole and installed on the roof of the building. The detected radio signal is processed and digitized by a sound card (Focusrite Scarlett 2i2), which is connected to a PC running under a Linux operating system. The electronics boxes, power supply and connecting cables are in an air-conditioned environment. The compact arrangement of devices still enables easy maintainability.
The VLF/LF system simultaneously measures the amplitude and phase of several transmitters (up to 20 channels are planned) with a chosen temporal resolution of 1 s. For the electric field antenna, as an outdoor element, it is important to have a hemispherical view; i.e., there are no or at least minimal shadowing effects due to the surrounding area and (intermittent) electromagnetic interference from the environment. This makes the site selection a crucial issue, in particular if the facility is located in an urban area, which in parallel enables benefits in terms of maintenance.
The proposed system has heritage from a predecessor facility (Schwingenschuh et al., 2011). It was possible to keep the VLF/LF measurement service without interruption for more than 10 years (Eichelberger et al., 2020), i.e., a full solar cycle. Short power line interruptions were bridged by an uninterruptible power supply (UPS).
Figure 3 displays VLF observations recorded by the new system in Graz (Austria). We have selected the observation of 20 March 2019 for which a solar flare C4.8 X-ray has been detected (see https://www.spaceweatherlive.com/en/solar-activity/top-50-solar-flares/year/2019.html, last access: 15 November 2023). In the top panel of Fig. 3 we show the variation of the amplitude (right vertical axis) and the phase (left vertical axis) versus the time in UT (horizontal axis) for the GBZ transmitter localized in Skelton (Great Britain) emitting at the frequency of 22.1 kHz. The middle panel shows the solar X-ray flux versus the time as recorded by Geostationary Operational Environmental Satellite (GOES). The bottom panel displays the TBB transmitter signal emitted from Bafa (Turkey) at a frequency of 26.7 kHz. The intermittent noisy variations of the transmitter signals (amplitude and phase) during daytime are local interferences because of the site location in an urban area. The great circle paths are of the order of 1540 and 1445 km between the Graz facility and the GBZ and TBB transmitters, respectively. It is important to note that the VLF signal enhancements of both transmitters (GBZ and TBB) around 12:00 UT coincide with the increase in the solar flare C4.8 X-ray recorded by GOES. Since the operation of the new system in 2019, preliminary results have been investigated, particularly those related to the VLF/LF propagation in the Earth's waveguide (Eichelberger et al., 2021) and also case studies of seismo-electromagnetic events based on combined observations from the China Seismo-Electromagnetic Satellite (CSES) (Zhang et al., 2020).
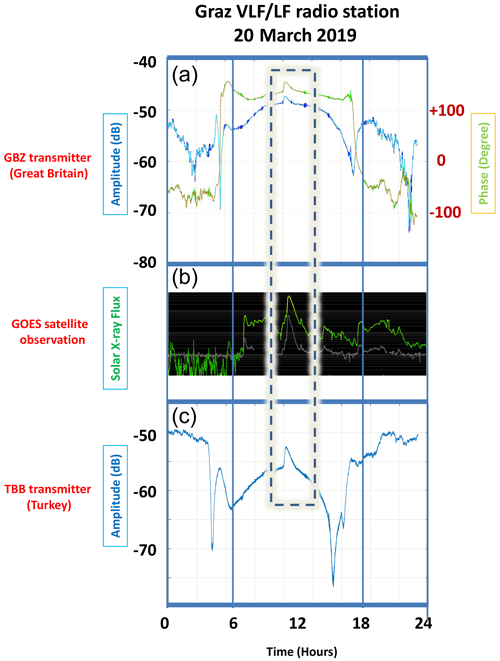
Figure 3VLF observations recorded on 20 March 2019 by the reception system (see Fig. 1). Panels (a) and (c) respectively display GBZ (Great Britain) and TBB (Turkey) transmitter signals detected by the Graz VLF/LF radio station. VLF signal enhancements of both transmitters (GBZ and TBB) around 12:00 UT coincide with the increase in the solar flare C4.8 X-ray (b). The dashed rectangle indicates the start and the end of this solar event as well as the corresponding observations in GBZ and TBB radio signals.
Our study is based on the observation of the variability the in amplitude and phase of the radio wave emitted by VLF/LF transmitters in order to determine a typical seismic signature. The wave properties of the transmitter signal will be affected during its propagation due to ionospheric disturbances above the EQ preparation zone (Hayakawa, 2015). The analysis of the received signal should allow characterizing and defining the “precursor signature” occurring on the ray path between the transmitter and the receiver stations. Then it is relevant to have the densest possible mesh of antennas. While the current network is localized in Europe via the International Network for Frontier Research on Earthquake Precursors (INFREP, Biagi et al., 2019), we propose a new location with two antennas in the Indian Ocean: the first one in the Southern Hemisphere (Réunion Island) and another one near the Equator (Sri Lanka). Figure 4 displays the location of the new receiving facilities and the geodesic paths towards some of the relevant transmitters, showing the importance of this new configuration. The Earth's seismic activity is mainly (but not exclusively) linked to tectonic activity and, in particular, to subduction zones where the lithosphere sinks into the terrestrial mantle. Thus many EQs occur at the boundary of convergent plates. A map of the location of convergent plate margins on Earth is displayed by Stern (2002, Fig. 1a).
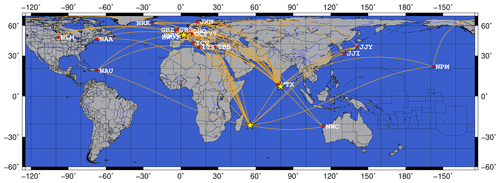
Figure 4The map shows the location (yellow stars) of the new VLF/LF receiving facilities in Guyancourt, Réunion and Sri Lanka together with important transmitter stations (red squares) and their corresponding great circle paths (orange lines). The Sri Lanka station is particularly useful to sample the area close to the Sunda subduction zone with large earthquakes via the path to the NWC transmitter (19.8 kHz) in Western Australia. The facility in Réunion enables a broad range of paths over East Africa.
Setting up an antenna on Réunion Island, in addition to benefiting from the scientific and technical infrastructure already existing on-site, will be particularly interesting for the study of seismicity and volcanism along the East African rift system, linked to the progression of the Somalian plate towards the African plate (see Bird, 2003, Fig. 18). Let us also note that the planned new VLF station in Réunion will lead to larger coverage of this region of the world and can be combined with other VLF networks in the Southern Hemisphere, like SAVNET in Brazil (Raulin et al., 2009).
Another VLF/LF facility shall be set up in Sri Lanka, as shown in Fig. 4. Due to its unique location, this island in the Indian Ocean captured the interest of many western nations in history. Also, its proximity to the Equator provides accessibility to the equatorial region where the so-called equatorial electrojet occurs (Forbes, 1981). The intended location of the VLF receiver is the University of Moratuwa, which is closer to the western edge of Sri Lanka.
It is important to note that Sri Lanka already hosts another low-frequency receiver from the World Wide Lightning Location Network (Dowden et al., 2002; Lay et al., 2004). Hence the University of Peradeniya, localized in the center of the country, currently holds a VLF receiver with magnetic loop antennas. Both systems in Moratuwa and Peradeniya cover the same frequency range but present different hardware configurations. Our proposed system uses a monopole electric antenna for signal reception, whereas the present VLF receiver at the University of Peradeniya has two magnetic loop antennas oriented along magnetic north–south and east–west directions. The goal of the magnetic loop antenna system is to study the electron and ion compositions of equatorial ionosphere and lightning observations (Maxworth et al., 2021). The combination of the two systems will help the calibration process and the possibility to cross-check natural and strong human-made signals. In addition to finding earthquake precursor signatures we will emphasize the performance of the two VLF/LF antenna configurations.
The installation of the two receivers in Réunion and Sri Lanka will be preceded by setting up (currently in progress) an antenna and its reception system on the LATMOS site in Guyancourt (near Paris) in order to finalize the data acquisition, processing and storage chain. It will also allow testing and validating the right functioning of the equipment, which will be the first node of this new VLF/LF network.
The data collected from our new network of VLF/LF receivers (Guyancourt, Réunion, Sri Lanka) are intended to join the International Network for Frontier Research on Earthquake Precursors (INFREP, Biagi et al., 2019). In addition, to better study the wave propagation in the ionosphere, observations from ground-based VLF/LF stations will be complemented by space observations, especially those collected by the China Seismo-Electromagnetic Satellite (CSES). This mission is the first Chinese satellite devoted to the investigation of ionospheric disturbances in connection with EQs (Shen et al., 2018). It was launched on 2 February 2018 and orbits at an altitude of ∼ 507 km at fixed local times: 02:00 and 14:00 LT. Investigations have been done about magnetic field variations measured at low altitude by CSES and ESA's Swarm satellites in connection with EQs (Schirninger et al., 2021). Boudjada et al. (2021) have performed a wave spectral analysis of the intensity variations of a VLF signal emitted by the ground-based NWC transmitter radio station (localized at North West Cape, Australia; see Fig. 4) observed by the electric field experiment (EFD) on board the CSES. Additionally, the data collected by our new VLF/LF network will also be combined with those from the ionospheric sounding instrument IONO on board the (CubeSat) nano-satellite INSPIRE-SAT 7 (Meftah et al., 2022), which was launched on 15 April 2023 in a Sun-synchronous orbit with descending node near 09:30 LT. It orbits at an altitude between 490 and 530 km. Magnetic field measurements along the orbit can also be used in addition to those from the other CubeSat UVSQ-SAT, the precursor nano-satellite of INSPIRE-SAT 7, launched on 24 January 2021 (Meftah et al., 2021).
In this paper, we present a new VLF/LF reception network to search for and study earthquake electromagnetic precursors. The proposed system will allow daily monitoring of transmitter signals in the VLF (3–30 kHz) and LF (30–300 kHz) frequency bands. Each individual facility consists of a monopole antenna, a preamplifier, a GPS receiver and a sound recorder card; it is identical to the UltraMSK system already in operation in Graz (Austria). At a first step, three antennas and their reception devices will be deployed in Guyancourt (France), Réunion (France) and Moratuwa (Sri Lanka). The collected data are intended to join the INFREP network and will be completed by space observations performed by the CSES as well as the INSPIRE-SAT 7 and UVSQ-SAT CubeSats, allowing the enhancement of the capability of earthquake electromagnetic precursor detections. Of course additional observational approaches and observables can be considered (Meng et al., 2019) for an integrated perspective in order to characterize atmospheric and ionospheric excitations related to natural hazard events. The actual status of seismo-electromagnetic investigations as evaluated and reviewed in recent papers (Eppelbaum, 2021; Chen et al., 2022) highlights the VLF/LF techniques and related methods in the framework of geophysical prospecting with a solid Earth background.
The UltraMSK code package is available for purchase at https://www.ultramsk.com (last access: 22 November 2023). It was developed by James Brundell from Dunedin, New Zealand. All details are given on the web page.
Data used in this work are available upon request to the corresponding author.
PHMG, ASM, MYB and HUE have co-written the paper. PHMG is the coordinator of the new antenna network and is supervising the installation of the new equipment. ASM is involved in setting up the VLF antenna in Sri Lanka. MYB, HUE and KS have implemented the UltraMSK reception facility in Graz. MYB carried out the study presented in Fig. 3, and HUE made the map shown in Fig. 4. MM is participating in the installation of the VLF antennas in France. PFB is the PI of the INFREP network. All co-authors reviewed the paper.
The contact author has declared that none of the authors has any competing interests.
Publisher's note: Copernicus Publications remains neutral with regard to jurisdictional claims made in the text, published maps, institutional affiliations, or any other geographical representation in this paper. While Copernicus Publications makes every effort to include appropriate place names, the final responsibility lies with the authors.
The map was created by the Generic Mapping Tool (GMT) software.
The first two VLF devices have been funded by the Programme National PNST of CNRS/INSU co-funded by CNES and CEA (grant no. AO INSU AA 2021).
This paper was edited by Lev Eppelbaum and reviewed by Jean-Pierre Raulin and one anonymous referee.
Biagi, P. F., Colella, R., Schiavulli, L., Ermini, A., Boudjada, M., Eichelberger, H., Schwingenschuh, K., Katzis, K., Contadakis, M. E., Skeberis, C., Moldovan, I. A., and Bezzeghoud, M.: The INFREP Network: Present Situation and Recent Results, Open Journal of Earthquake Research, 8, 101–115, https://doi.org/10.4236/ojer.2019.82007, 2019. a, b, c
Bird, P.: An updated digital model of plate boundaries, Geochem. Geophys. Geosy., 4, 1027, https://doi.org/10.1029/2001GC000252, 2003. a
Boudjada, M. Y., Biagi, P. F., Al-Haddad, E., Galopeau, P. H. M., Besser, B., Wolbang, D., Prattes, G., Eichelberger, H., Stangle, G., Parrot, M., and Schwingenschuh, K.: Reception conditions of low frequency (LF) transmitter signals onboard DEMETER micro-satellite, Phys. Chem. Earth, 102, 70–79, https://doi.org/10.1016/j.pce.2016.07.006, 2017. a
Boudjada, M. Y., Eichelberger, H. U., Zhang, X., Magnes, W., Denisenko, V., Pollinger, A., Galopeau, P. H. M., Schwingenschuh, K., and Besser, B.: Analysis of ground-based very low frequency signal recorded onboard CSES satellite, in: 2021 Kleinheubach Conference, Miltenberg, Germany, 28–30 September 2021, 1–3, https://doi.org/10.23919/IEEECONF54431.2021.9598373, 2021. a
Bowman, D. D., Ouillon, G., Sammis, C. G., Sornette, A., and Sornette, D.: An observational test of the critical earthquake concept, J. Geophys. Res., 103, 24359–24372, https://doi.org/10.1029/98JB00792, 1998. a
Chen, H., Han, P., and Hattori, K.: Recent advances and challenges in the seismo-electromagnetic study: A brief review, Remote Sens., 14, 5893, https://doi.org/10.3390/rs14225893, 2022. a
Cicerone, R. D., Ebel, J. E., and Britton, J.: A systematic compilation of earthquake precursors, Tectonophysics, 476, 371–396, https://doi.org/10.1016/j.tecto.2009.06.008, 2009. a
Clilverd, M. A., Rodger, C. J., Thomson, N. R., Brundell, J. B., Ulich, T., Lichtenberger, J., Cobbett, N., Collier, A. B., Menk, F. W., Seppälä, A., Verronen, P. T., and Turunen, E.: Remote sensing space weather events: Antarctic-Arctic Radiation-belt (Dynamic) Deposition-VLF Atmospheric Research Konsortium network, Space Weather, 7, S04001, https://doi.org/10.1029/2008SW000412, 2009. a
De Santis, A., Abbattista, C., Alfonsi, L., Amoruso, L., Campuzano, S. A., Carbone, M., Cesaroni, C., Cianchini, G., De Franceschi, G., De Santis, A., Di Giovambattista, R., Marchetti, D., Martino, L., Perrone, L., Piscini, A., Rainone, M. L., Soldani, M., Spogli, L., and Santoro, F.: Geosystemics view of earthquakes, Entropy, 21, 412, https://doi.org/10.3390/e21040412, 2019. a
Dobrovolsky, I. P., Zubkov, S. I., and Myachkin, V. I.: Estimation of the size of earthquake preparation zones, Pure Appl. Geophys., 117, 1025–1044, https://doi.org/10.1007/BF00876083, 1979. a
Dowden, R. L., Brundell, J. B., and Rodger, C. J.: VLF lightning location by time of group arrival (TOGA) at multiple sites, J. Atmos. Sol.-Terr. Phy., 64, 817–830, https://doi.org/10.1016/S1364-6826(02)00085-8, 2002. a
Eichelberger, H., Schwingenschuh, K., Boudjada, M. Y., Besser, B. P., Wolbang, D., Rozhnoi, A., Solovieva, M., Biagi, P. F., Stachel, M., Aydogar, Ö., Muck, C., Grill, C., and Jernej, I.: Characterization of sub-ionospheric VLF/LF waveguides for seismic event studies during solar minimum, EGU General Assembly 2020, Vienna, Austria, 4–8 May 2020, https://doi.org/10.5194/egusphere-egu2020-15760, 2020. a
Eichelberger, H., Boudjada, M. Y., Schwingenschuh, K., Galopeau, P., Rozhnoi, A., Solovieva, M., Biagi, P., Fedun, V., and Besser, B. P.: Sub-ionospheric VLF/LF waveguide anomalies over Europe, in: Proceedings of the XXXIVth URSI General Assembly in Rome, Fr–GHE–AM1–1, https://www.ursi.org/proceedings/procGA21/papers/URSIGASS2021-Fr-GHE-AM1-1.pdf (last access: 15 November 2023), 2021. a
Eppelbaum, L. V.: VLF-method of geophysical prospecting: A non-conventional system of processing and interpretation (implementation in the Caucasian ore deposits), ANAS Transactions, Earth Sciences, 2/2021, 16–38, https://doi.org/10.33677/ggianas20210200060, 2021. a
Forbes, J. M.: The Equatorial Electrojet, Rev. Geophys. Space Ge., 19, 469–504, https://doi.org/10.1029/RG019i003p00469, 1981. a
Hayakawa, M.: VLF/LF Techniques, Chap. 5, John Wiley & Sons, Ltd., 139–197, https://doi.org/10.1002/9781118770368.ch5, 2015. a, b, c
Hayakawa, M. and Hobara, Y.: Current status of seismo-electromagnetics for short-term earthquake prediction, Geomat. Nat. Haz. Risk, 1, 115–155, https://doi.org/10.1080/19475705.2010.486933, 2010. a
Hayakawa, M., Molchanov, O. A., Ondoh, T., and Kawai, E.: The precursory signature effect of the Kobe earthquake on VLF subionospheric signals, J. Comm. Res. Lab. Tokyo, 43, 169–180, 1996. a
Hayakawa, M., Raulin, J. P., Kasahara, Y., Bertoni, F. C. P., Hobara, Y., and Guevara-Day, W.: Ionospheric perturbations in possible association with the 2010 Haiti earthquake, as based on medium-distance subionospheric VLF propagation data, Nat. Hazards Earth Syst. Sci., 11, 513–518, https://doi.org/10.5194/nhess-11-513-2011, 2011. a
Keilis-Borok, V.: Earthquake prediction: State-of-the-art and emerging possibilities, Annu. Rev. Earth Pl. Sc., 30, 1–33, https://doi.org/10.1146/annurev.earth.30.100301.083856, 2002. a
Lay, E. H., Holzworth, R. H., Rodger, C. J., Thomas, J. N., Pinto, O., and Dowden, R. L.: WWLL global lightning detection system: Regional validation study in Brazil, Geophys. Res. Lett., 31, L03102, https://doi.org/10.1029/2003GL018882, 2004. a
Maxworth, A., Lichtenberger, J., Liyanage, P., and Gołkowski, M.: First results from the ELF/VLF receiver in Sri Lanka, in: Proceedings of the XXXIVth URSI General Assembly in Rome, Fr–H01–AM2–3, https://www.ursi.org/proceedings/procGA21/papers/URSIGASS2021-Fr-H01-AM2-3.pdf (last access: 15 November 2023), 2021. a
Maxworth, A. S., Gołkowski, M., Cohen, M. B., Moore, R. C., Chorsi, H. T., Gedney, S. D., and Jacobs, R.: Multistation observations of the azimuth, polarization, and frequency dependence of ELF/VLF waves generated by electrojet modulation, Radio Sci., 50, 1008–1026, https://doi.org/10.1002/2015RS005683, 2015. a
Meftah, M., Boutéraon, T., Dufour, C., Hauchecorne, A., Keckhut, P., Finance, A., Bekki, S., Abbaki, S., Bertran, E., Damé, L., Engler, J.-L., Galopeau, P., Gilbert, P., Lapauw, L., Sarkissian, A., Vieau, A.-J., Lacroix, P., Caignard, N., Arrateig, X., Hembise Fanton d’Andon, O., Mangin, A., Carta, J.-P., Boust, F., Mahé, M., and Mercier, C.: The UVSQ-SAT/INSPIRESat-5 CubeSat mission: First in-orbit measurements of the Earth’s outgoing radiation, Remote Sens., 13, 1449, https://doi.org/10.3390/rs13081449, 2021. a
Meftah, M., Boust, F., Keckhut, P., Sarkissian, A., Boutéraon, T., Bekki, S., Damé, L., Galopeau, P., Hauchecorne, A., Dufour, C., Finance, A., Vieau, A.-J., Bertran, E., Gilbert, P., Caignard, N., Dias, C., Engler, J.-L., Lacroix, P., Grossel, K., Rannou, V., Saillant, S., Avelino, Y., Azoulay, B., Brand, C., Dominguez, C., Haasz, A., Paskeviciute, A., Segura, K., Maso, P., Ancelin, S., Mercier, C., Stee, V., Mangin, A., Bolsée, D., and Billard, C.: INSPIRE-SAT 7, a second CubeSat to measure the Earth's energy budget and to probe the ionosphere, Remote Sens., 14, 186, https://doi.org/10.3390/rs14010186, 2022. a
Meng, X., Vergados, P., Komjathy, A., and Verkhoglyadova, O.: Upper atmospheric responses to surface disturbances: An observational perspective, Radio Sci., 54, 1076–1098, https://doi.org/10.1029/2019RS006858, 2019. a
Molchanov, O., Rozhnoi, A., Solovieva, M., Akentieva, O., Berthelier, J. J., Parrot, M., Lefeuvre, F., Biagi, P. F., Castellana, L., and Hayakawa, M.: Global diagnostics of the ionospheric perturbations related to the seismic activity using the VLF radio signals collected on the DEMETER satellite, Nat. Hazards Earth Syst. Sci., 6, 745–753, https://doi.org/10.5194/nhess-6-745-2006, 2006. a
Nina, A., Biagi, P. F., Mitrović, S. T., Pulinets, S., Nico, G., Radovanović, M., and Popović, L. Č.: Reduction of the VLF signal phase noise before earthquakes, Atmosphere, 12, 444, https://doi.org/10.3390/atmos12040444, 2021. a
Parrot, M.: DEMETER Satellite and Detection of Earthquake Signals, Chap. 6, 115–138, CRC Press, Boca Raton, https://doi.org/10.1201/9781315166841, 2018. a
Raulin, J.-P., Correia de Matos David, P., Hadano, R., Saraiva, A. C. V., Correia, E., and Kaufmann, P.: The South America VLF NETwork (SAVNET), Earth, Moon Planets, 104, 247–261, https://doi.org/10.1007/s11038-008-9269-4, 2009. a, b
Samanes, J. E., Raulin, J.-P., Macotela, E. L., and Guevara Day, W. R.: Estimating the VLF modal interference distance using the South America VLF Network (SAVNET), Radio Sci., 50, 122–129, https://doi.org/10.1002/2014RS005582, 2015. a
Schirninger, C., Eichelberger, H. U., Magnes, W., Boudjada, M. Y., Schwingenschuh, K., Pollinger, A., Besser, B. P., Biagi, P. F., Solovieva, M., Wang, J., Cheng, B., Zhou, B., Shen, X., Delva, M., and Lammegger, R.: Satellite measured ionospheric magnetic field variations over natural hazards sites, Remote Sens., 13, 2360, https://doi.org/10.3390/rs13122360, 2021. a
Schwingenschuh, K., Prattes, G., Besser, B. P., Močnik, K., Stachel, M., Aydogar, Ö., Jernej, I., Boudjada, M. Y., Stangl, G., Rozhnoi, A., Solovieva, M., Biagi, P. F., Hayakawa, M., and Eichelberger, H. U.: The Graz seismo-electromagnetic VLF facility, Nat. Hazards Earth Syst. Sci., 11, 1121–1127, https://doi.org/10.5194/nhess-11-1121-2011, 2011. a
Shen, X., Zhang, X., Yuan, S., Wang, L., Cao, J., Huang, J., Zhu, X., Piergiorgio, P., and Dai, J.: The state-of-the-art of the China Seismo-Electromagnetic Satellite mission, Sci. China Technol. Sc., 61, 634–642, https://doi.org/10.1007/s11431-018-9242-0, 2018. a
Stern, R. J.: Subduction zones, Rev. Geophys., 40, 1012, https://doi.org/10.1029/2001RG000108, 2002. a
Yoshida, M., Yamauchi, T., Horie, T., and Hayakawa, M.: On the generation mechanism of terminator times in subionospheric VLF/LF propagation and its possible application to seismogenic effects, Nat. Hazards Earth Syst. Sci., 8, 129–134, https://doi.org/10.5194/nhess-8-129-2008, 2008. a
Zhang, X., Wang, Y., Boudjada, M. Y., Liu, J., Magnes, W., Zhou, Y., and Du, X.: Multi-experiment observations of ionospheric disturbances as precursory effects of the Indonesian Ms6.9 earthquake on August 05, 2018, Remote Sens., 12, 4050, https://doi.org/10.3390/rs12244050, 2020. a